Last time
I wrote this
review on what roles parasites might play in ecology and evolution. Here I’m
back to summarize the results of a new paper, exploring whether parasites can
contribute to a process which is right at the intersection of ecology and
evolution: ecological speciation. For this purpose we studied the colourful
cichlids from Lake Tanganyika, one of the prime examples of explosive adaptive
radiations. We made this choice because parasites are often ignored in
explaining cichlid radiations, even though habitat shifts and diet shifts
(i.e., two commonly assumed drivers of cichlid radiations) are inevitably
associated with shifts in infection risk.
Lake
Tanganyika is much older and deeper than the other Great East-African Lakes
(e.g. Lake Victoria or Lake Malawi), and lake level fluctuations are thought to
influence speciation processes by bringing fish populations into episodes of
isolation and secondary contact. We assumed that this not only happens with the
fish, but also with their parasite communities. The result would be that
different fish populations end up with divergent parasite communities. Local
differences in extinction risk and species sorting and local adaptation of the
parasites would amplify these differences even more. In fact, under this
scenario, major habitat and diet shifts would not even be required to observe
differences in parasitism between populations and species.
To test
this expectation, we investigated the rock-dwelling
cichlid Tropheus. This species lives on
algae which it scrapes from the rocks. Allopatric populations are highly
diverse in colour, and there are more than 100 colour morphs across the lake.
Despite this diversity, all populations are ecomorphologically equivalent – i.e.
they all live in habitats with rocky substrate, they all scrape algae, and they
all look similar for morphological traits other than colour. Colour itself is
believed to play a major role in mate recognition in Tropheus, and hence might be involved in the evolution of
reproductive barriers, like when populations of different colours come into
secondary contact. However, there is no direct evidence that colour matters for
mate choice in Tropheus, and even when it does matter, it might be that is marking
something else, such as the condition of the fish or local adaptation – for
instance, resistance to parasites.
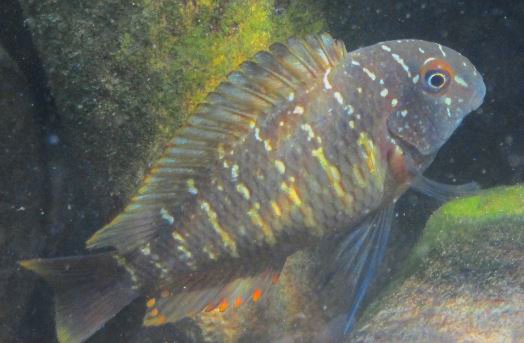
Tropheus sp.
hanging around at Kalambo Lodge, Lake Tanganyika, Zambia (Photo: Pascal Hablützel).
With a 700
km long lake north to south and hardly any of the parasite species described,
starting this project on Lake Tanganyika back in 2010 was kind of a challenge.
In search of adventure we decided to start at the Congo side of the lake. For
this purpose we rented the “Primus”, a
shiny, blue 30 feet vessel which otherwise does not transport scientists, but
Primus, the local beer. The vessel came with a complete crew, including a
captain, a cook, the helper of the cook, two lookouts, and three local
scientists of the “Centre de Recherche en Hydrobiologie” from Uvira. The idea
behind all this logistical support was to sail from Uvira in the North to Moba,
450 km south (and back). The previous ichthyological expedition of this scale
dated from the 1940’s, so a general update on fish (and parasite) diversity
seemed like a useful thing to do. Despite a number of limitations, including barely
enough horse power to sail faster than 10 km/h, rebellion armies hindering us access
to land, the official army eager to inspect our boat for Primus beer, too strong anti-malaria pills, and the
occasional thunderstorm, we completed the round-trip in about five weeks.
Our
vessel, the “Primus”, not exactly well camouflaged for rebellion armies, at
it’s top speed of 10 km/h (Photo:
Maarten Van Steenberge)
Sub-optimal
(top) vs. optimal (bottom) sailing conditions on Lake Tanganyika (Photos: Joost
Raeymaekers)
Even
though the expedition was a great success and refreshed our knowledge of fish
diversity (see here),
we failed on one point: we hardly found any parasites in the Tropheus populations. As parasites are
kind of essential for a project on parasite-driven adaptation and speciation, we
decided to try again in 2011 and 2012 in another season and in a logistically
less challenging part of the Lake: the Zambian shore. We selected eight Tropheus populations from five different
colour morphs along the shore, and screened them for parasites. This time there
were lots of parasites, and it turned out that different populations do harbour
consistently different parasite communities - especially among populations from
different colour morphs.
Top:
Lake Tanganyika with its colourful Tropheus
populations. Middle: STRUCTURE plot
showing that the red, blue, light-olive and dark-olive colour morphs are
isolated genetically. Bottom:
neighbouring Tropheus populations show
differences in infection parameters for various groups of parasites. The black
bars (‘barriers’) indicate significant differences in a single year (dashed
bars) or in two consecutive years (full bars). Chilanga and Linangu in the west
is crocodile country, and we decided to scare them only in the first field year.
These differences
in diversity and magnitude of infection imply that fragmented Tropheus populations experience divergent
parasite selection pressures. Parasites might therefore contribute to
adaptation and reproductive isolation in Tropheus.
It is not yet clear whether this is really the case, or whether parasite
communities just co-vary with something else. So, the next step is to test
whether Tropheus populations are actually
adapted to local parasites communities. If so, several pathways of how
parasites could induce or contribute to reproductive isolation could be tested.
Cichlidogyrus,
a flatworm parasite specific to cichlids, and a close cousin of Gyrodactylus. This gill parasite is one
of the most common parasites we observed – usually more than 80% of the fish
are infected (Photo: Maarten Vanhove).
Of course,
parasites represent only one component of the ecosystem, and allopatric
populations of cichlids differ in many other known and unknown parameters. In
addition, parasites are very diverse, and cannot be considered as a single
selection pressure. The parasites investigated in our study included the community
of metazoan macroparasites, which differ dramatically in life cycle (some
depend on a single host, some also on intermediate and final hosts) and host
specificity (hosts during a given life stage of the parasite might belong to
one or several hosts). As a result, their impact on host ecology and evolution might
vary quite a bit. Given this complexity, there might be several opportunities
for eco-evolutionary dynamics. For instance, one parasite species might be strongly influencing host biology
and contribute to parasite-driven speciation, while another parasite species
might only feel the consequences of that. A related complexity is that part of
the parasite community can disperse with their hosts. Hence, strong dispersing
cichlid species might homogenize their own parasite communities across
habitats, and thus, parasite communities might not impose divergent selection
at all. We therefore also started comparing immunogenetic adaptation in Tropheus, which is a weak disperser,
with Simochromis, a related cichlid
species which is a very strong disperser. We will be back on this blog to report
the results.
Raeymaekers
JAM, Hablützel PI, Grégoir AF, Bamps J, Roose AK, Vanhove MPM, Van Steenberge
M, Pariselle A, Huyse T, Snoeks J & Volckaert FAM (2013). Contrasting
parasite communities among allopatric colour morphs of the Lake Tanganyika
cichlid Tropheus. BMC Evolutionary Biology 13, 41.
The
ever-enthusiastic people of Congo (Photo: Joost Raeymaekers).